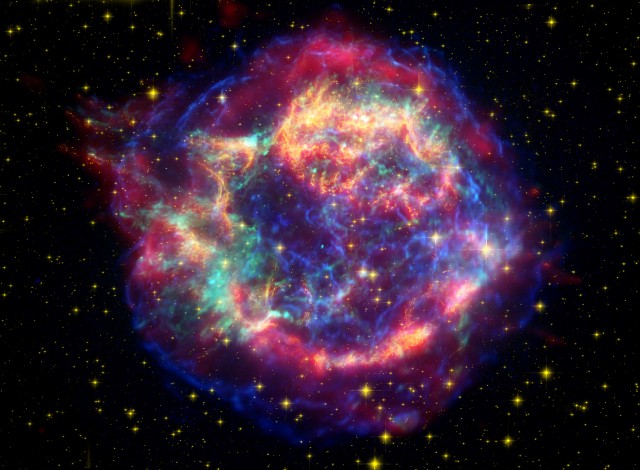
NASA/JPL-Caltech/ O. Krause (Steward Observatory)
Since the discovery of neutron stars, researchers have used their unusual properties to investigate our universe. The super-dense remnants of stellar explosions, neutron stars pack a mass greater than that of the Sun into a ball about the width of San Francisco. A single cup of this stardust would weigh about as much as Mount Everest.
These strange celestial objects can warn us of distant perturbations in the fabric of spacetime, teach us about the formation of elements, and unravel the secrets of how gravity and particle physics work in some of the universe’s most extreme conditions.
“They are at the center of many open questions in astronomy and astrophysics,” says astrophysicist Vanessa Graber of the Institute of Space Sciences in Barcelona.
But to accurately interpret some of the signals from the neutron stars, researchers must first understand what’s going on inside. They have their premonitions, but experimenting directly on a neutron star is out of the question. So scientists need another way to test their theories. The behavior of matter in such a super-dense object is so complicated that even computer simulations are not up to the task. But researchers think they may have found a solution: a terrestrial analogue.
Although young neutron stars can have temperatures of millions of degrees inside them, neutrons are considered “cold” by an important energetic measure. Physicists think this is a feature they can use to study the inner workings of neutron stars. Instead of looking at the sky, researchers look into clouds of ultra-cold atoms created in laboratories here on Earth. And that could help them finally answer some long-standing questions about these puzzling objects.
Peculiarities in space
The existence of neutron stars was first proposed in 1934, two years after the discovery of the neutron itself, when astronomers Walter Baade and Fritz Zwicky wondered if a celestial body composed entirely of neutrons could persist after a supernova explosion. While they didn’t get all the details right, their general idea is now widely accepted.
Stars feed themselves by fusing the nuclei of lighter atoms into those of heavier atoms. But when the stars run out of lighter atoms, nuclear fusion stops and there is no external pressure to fight against internal gravity. The core collapses and the outer layer of the star hurtles inward. When this layer hits the dense core, it bounces off and explodes outward, creating a supernova. The dense core that remains afterwards is a neutron star.
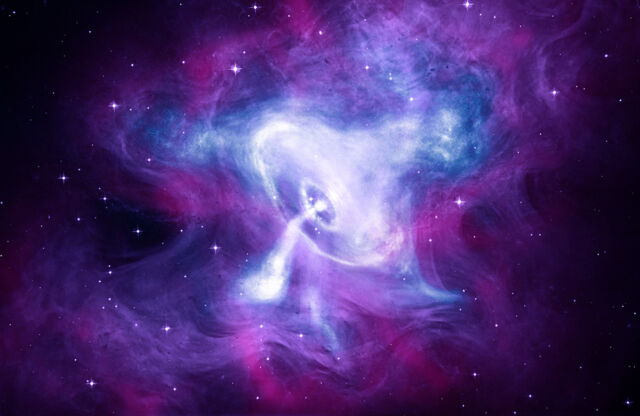
CREDIT: NASA: X-RAY: CHANDRA (CXC), OPTICAL: HUBBLE (STSCI), INFRARED: SPITZER (JPL-CALTECH)
It wasn’t until the 1960s that the hypothetical Zwicky and Baade neutron stars were finally discovered. Radio astronomer Jocelyn Bell Burnell noticed a strange, regularly pulsed radio wave signal coming from space while working as a graduate student at Cambridge University. She detected something that had never been seen before: a special kind of neutron star called a pulsar, which flashes beams of radiation at regular intervals as it rotates, like a lighthouse. (Her advisor, along with the observatory’s director — but not Bell Burnell — later received the Nobel Prize for the discovery.)
Since then, thousands of neutron stars have been discovered. As some of the densest, highest-pressured objects in the universe, neutron stars could help us learn what happens to matter at extremely high densities. Understanding their structure and the behavior of the neutron matter of which they are composed is of paramount importance to physicists.
Scientists already know that the neutrons, protons and other subatomic particles that make up a neutron star arrange themselves differently depending on where they are in the star. In certain sections, they pack tightly like water molecules in a block of ice. In others, they flow and swirl like a frictionless fluid. But exactly where the transition takes place and how the different phases of matter behave, physicists are not sure.
A super-dense star born from a nuclear fireball seems at first glance to have little in common with a dilute cloud of ultracold particles. But they may share at least one useful characteristic: They both lie below a threshold known as the Fermi temperature that depends on — and is calculated from — the matter each system is made of. A system well above this temperature will behave largely according to the laws of classical physics; if it is far below it, its behavior will be determined by quantum mechanics. Certain ultracold gases and material from neutron stars can both be well below their Fermi temperatures and therefore act similarly, says Christopher Pethick, a theoretical physicist at the Niels Bohr Institute in Copenhagen and co-author of an early survey of neutron stars in the 1975 annual issue. Nuclear Science Reviewe.
Matter below its Fermi temperature can obey remarkably universal laws. This universality means that while we don’t have easy access to multi-million-degree neutron star matter, we can learn something of its behavior by experimenting with ultracold gases that can be created and manipulated in laboratory vacuum chambers on Earth, says theoretical astrophysicist James Lattimer of Stony Brook. University of New York, author of a summary of the science of nuclear matter in the 2012 Annual Review of Nuclear and Particle Science.
Of particular interest to Lattimer is a theoretical state called a unit gas. A gas is unitary when the sphere of influence of each of its particles becomes infinite, meaning that they would affect each other no matter how far apart they are. This is impossible in reality, but ultracold atomic clouds can get close – and so can the matter in neutron stars. “It’s similar to a unitary gas,” says Lattimer, “but it’s not a perfect unitary gas.”