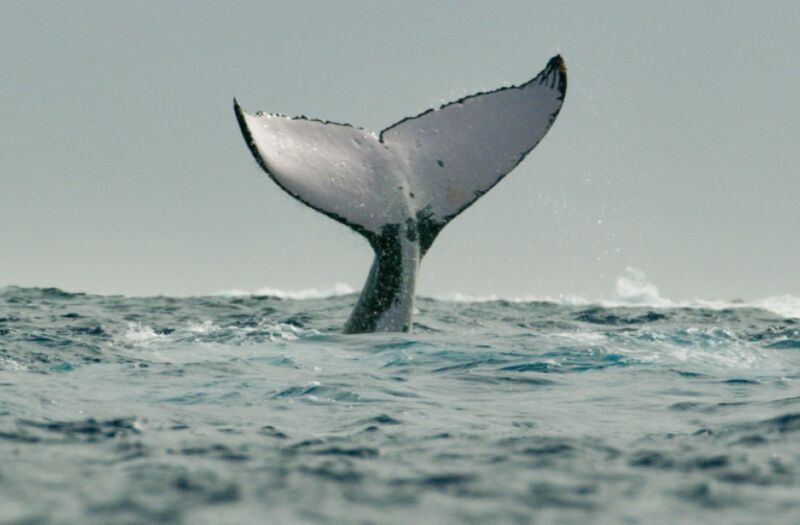
Hayes Baxley/National Geographic for Disney+
About 400 million years ago, the ancestor of all four-limbed creatures took its first steps on dry land. Fast-forward about 350 million years, and a descendant of these early landlubbers did a U-turn: He waded back into the water. In time, the back-to-the-sea creatures would spawn animals vastly different from their land-trotting relatives: they became the magnificent whales, dolphins, and porpoises that glide the oceans today.
Going back to aquatic life was a drastic step that would change the animals inside and out, over the span of about 10 million years — the blink of an eye in evolutionary terms. Members of this group, now called cetaceans, dropped their hind legs for powerful flukes and lost almost all of their hair. For decades, their bizarre body plans puzzled paleontologists, who speculated they might have evolved from creatures as varied as marine reptiles, seals, marsupials like kangaroos, and even a now-extinct group of wolf-like carnivores.
“The cetaceans are generally the most peculiar and anomalous mammals,” wrote a scientist in 1945.
Then, in the late 1990s, genetic data confirmed that whales were part of the same evolutionary lineage that spawned cows, pigs and camels — a branch called Artiodactyla. Fossils from modern-day India and Pakistan later formed that family tree, identifying cetaceans’ closest ancient relatives as small, wading deer-like creatures.
But their body plans are just the beginning of cetacean weirdness. To survive in the sea, they also had to make internal adjustments, changing their blood, saliva, lungs and skin. Many of those changes aren’t apparent in fossils, and cetaceans aren’t easy to study in the lab. Instead, it was once again genetics that brought them to light.
With increasing availability of cetacean genomes, geneticists can now search for the molecular changes that accompanied the transition from back to water. While it’s impossible to be sure of the influence of any particular mutation, scientists suspect that many of the mutations they see correspond to adaptations that allow cetaceans to dive and thrive in the deep blue sea.
Dive into the depths
The first cetaceans lost much more than legs when they went back to the water: whole genes stopped working. In the huge book of genetic letters that make up a genome, these defunct genes are among the easiest changes to detect. They stand out as a garbled or fragmented sentence and no longer code for a complete protein.
Such a loss can happen in two ways. Perhaps having a certain gene was somehow detrimental to cetaceans, so animals that lost it gained a survival advantage. Or it could be a “use it or lose it” situation, says genomicist Michael Hiller of the Senckenberg Research Institute in Frankfurt, Germany. If the gene had no function in the water, it would randomly accumulate mutations and the animals would be no worse off if it stopped functioning.
Hiller and colleagues dove into the back-to-water transition by comparing the genomes of four cetaceans — dolphin, killer whale, sperm whale and minke whale — with those of 55 land mammals plus a manatee, a walrus and the Weddell seal. About 85 genes stopped working as cetacean ancestors adapted to the sea, the team reported Scientific progress in 2019. In many cases, Hiller says, they could guess why those genes became defunct.
For example, cetaceans no longer possess a particular gene:SLC4A9—involved in saliva production. That makes sense: what good is spitting if your mouth is already full of water?
Cetaceans also lost four genes involved in the synthesis of and response to melatonin, a hormone that regulates sleep. The ancestors of whales probably discovered fairly quickly that they couldn’t come up to breathe if they shut down their brains for hours on end. Modern cetaceans sleep one hemisphere at a time, while the other hemisphere remains alert. “If you’re not having the normal sleep as we know it, then you probably don’t need melatonin,” says Hiller.
The long time whales have to hold their breath to dive and hunt also seems to have stimulated genetic changes. Deep diving, as divers know, means that small nitrogen bubbles can form in the blood and seed clots – something that was probably harmful to early cetaceans. Coincidentally, two genes (F12 and KLKB1) that normally help initiate blood clotting no longer work in cetaceans, likely lowering this risk. The rest of the clotting mechanism remains intact, so that whales and dolphins can still seal injuries.
Another lost gene – and this surprised Hiller – codes for an enzyme that repairs damaged DNA. He thinks this change also has to do with deep diving. When cetaceans surface to breathe, oxygen suddenly rushes into their bloodstream, and as a result, reactive oxygen molecules that can break apart DNA. The missing enzyme – DNA polymerase mu – normally repairs this kind of damage, but does so sloppily, often leaving behind mutations. Other enzymes are more accurate. Perhaps, Hiller thinks, mu was just too sloppy for the cetacean lifestyle, unable to handle the amount of reactive oxygen molecules produced by the constant diving and surfacing. Dropping the imprecise enzyme and leaving the repair to more accurate ones that cetaceans also possess makes it more likely that oxygen damage has been properly repaired.
Cetaceans are not the only mammals that returned to the water, and genetic losses in other aquatic mammals often parallel those in whales and dolphins. For example, both cetaceans and manatees have a gene called deactivated MMP12, which normally breaks down the stretchable lung protein elastin. Perhaps that deactivation helped both groups of animals develop highly elastic lungs, allowing them to rapidly exhale and inhale about 90 percent of their lung volume when they surface.
However, deep adjustments aren’t just about loss. One notable gain is in the gene that contains instructions for myoglobin, a protein that supplies oxygen to muscles. Scientists have examined myoglobin genes in diving animals, from tiny water shrews to giant whales, and discovered a pattern: In many divers, the surface of the protein has a more positive charge. That would cause the myoglobin molecules to repel each other like two north magnets. This, researchers suspect, allows diving mammals to maintain high concentrations of myoglobin without the proteins clumping together, and thus high concentrations of muscle oxygen while diving.