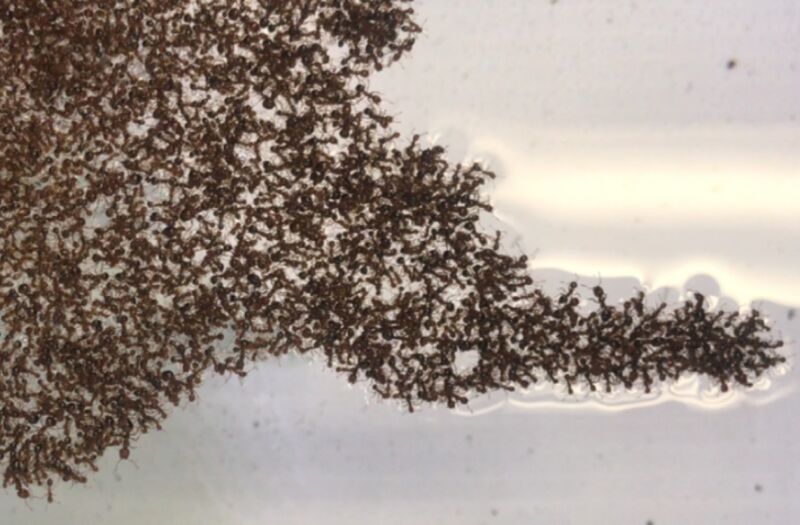
Vernerey Research Group/CU Boulder
Fire ants are a textbook example of collective behavior, able to behave as individuals and also coalesce to form floating rafts in response to flooding. Now, a few mechanical engineers at the University of Colorado, Boulder, have identified some simple rules that seem to govern how floating fire ant rafts contract and expand their shape over time, according to a new paper published in the journal PLOS Computational Biology. The hope is that by better understanding the simple rules that underlie fire ant behavior, they can develop better algorithms that control how swarms of robots interact with one another.
It’s not a matter of brain power or careful planning. “This behavior could essentially occur spontaneously,” said study co-author Robert Wagner. “There doesn’t necessarily have to be centralized decision-making by the ants.” Indeed, “Lone ants are not as smart as one might think, but together they become very intelligent and resilient communities,” said study co-author Franck Vernerey.
As we reported before, a pair of ants that are far apart behave like individual ants. But pack enough of them close together, and they behave more like a single unit, with both solid and liquid properties. They can form rafts or towers, and you can even pour them like a liquid from a teapot. Fire ants also excel at regulating their own traffic flow.
Every single ant has some degree of hydrophobia – the ability to repel water – and this trait is enhanced when they mate together and weave their bodies like a waterproof fabric. They collect all the eggs, make their way to the surface through their tunnels in the nest, and as the floodwaters rise, they chew each other’s bodies with their jaws and claws, until a flat, raft-like structure forms, with each ant acting as an individual molecule in a material, for example grains of sand in a sand heap.
The ants can achieve this in less than 100 seconds. In addition, the ant raft is “self-healing”: it is robust enough that if it loses an ant here and there, the overall structure can remain stable and intact, even for months. In short, the ant raft is a superorganism.
In 2019, researchers at Georgia Tech demonstrated that fire ants can actively sense changes in forces acting on the raft under different fluid conditions and adjust their behavior accordingly to maintain raft stability. For example, with a shear force, the surface of the raft was much smaller than if the ants experienced only centrifugal force. Ants experience the latter regardless of where they are in the ant raft, while only the ants at the border experience the strongest shear. The scientists hypothesized that the smaller rafts are the result of ants trying to avoid being at the borders, minimizing surface area in the process.
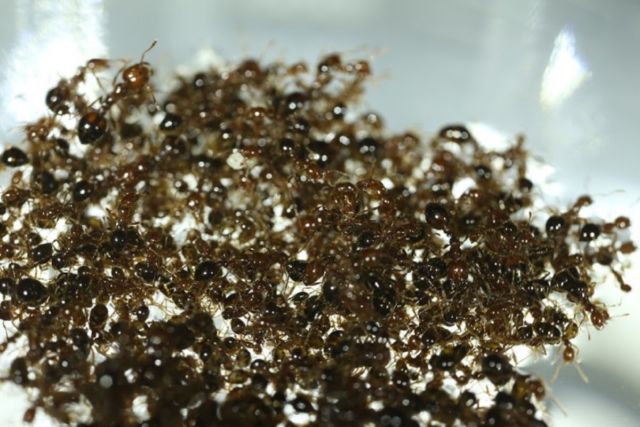
Hungtang Ko
The Georgia Tech team also noted that fire ants in a raft explore more when the raft is stationary — usually spreading horizontally, but also vertically, and building temporary tower-like structures in hopes of finding a hanging branch to grab onto to dry again. country. There will be much less exploratory behavior if the ant raft rotates in response to centrifugal or shear forces.
Vernerey and Wagner’s new research builds on a study they published last year. They conducted experiments by dropping hordes of fire ants into a bucket of water with a plastic vertical rod in the center, then followed the raft-building behavior of the ants for the next eight hours. The idea was to observe how the rafts evolved over time. They noticed that the rafts did not stay in the same shape. Sometimes the structures were compressed into tight circles of ants. Other times, the ants started fanning out to form bridge-like extensions, sometimes using them to escape the containers, suggesting the behavior could serve an evolutionary advantage.
The duo were fascinated by how the ants achieved those shape changes through a process they called “tredmilling.” The rafts are essentially made up of two different layers. Ants on the bottom tier serve a structural purpose and form the stable base of the raft. But the ants on the top layer move freely over the connected bodies of their brethren in the bottom layer. Sometimes ants move from the bottom to the top tier, or from the top to the bottom tier in a cycle Wagner calls “a donut-shaped treadmill.”
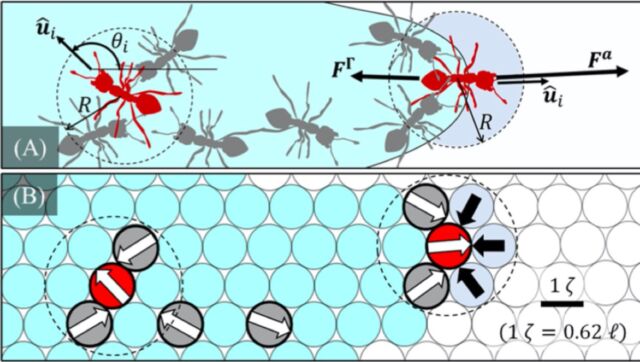
Wagner & Vernerey, 2022
Vernerey and Wagner wanted to determine whether this walking behavior was a conscious decision by the ants, or whether it arose spontaneously. So they created a series of agent-based models consisting of 2000 particles (“agents) representing each individual ant, confined to a lattice of water nodes. One population of agent-ants (shown in cyan) formed the basic structural network; other agent-ants (shown in red) were free to move on top of them.
The ants were programmed to follow a simple set of rules, such as avoiding collisions with other ants and not falling into the water (the “rule of edge deposition”). Then they let the simulations play. And the simulated ants behaved just like their real-life counterparts.
For example, when active agent ants reached the edge of the raft and came into contact with water, they avoided entering the water unless forced to do so by neighboring active agent ants – and then only if there were sufficient structural support ants to to grab. The simulations also showed bridge-like projections forming spontaneously, and the researchers were able to link those formations to the relative activity of the ants. The more active the ants were, the more likely protrusions would form.
“The ants on the ends of these protrusions are pushed almost off the edge into the water, leading to a runaway effect,” Wagner said. It’s possible these protrusions are a way for the fire ants on a raft to survey their environment, perhaps looking for a log or dry land.
“While cue factors such as pheromones have not been ruled out and should be tested in future experimental studies, this model generally provides local mechanisms by which fire ants can reach treadmills and protrusions without centralized control or deliberate intent,” the authors concluded. That said, they recognize that this is a homogenized model and that there will likely be more than one set of rules governing treadmill behavior and the appearance of protrusions — another future focus of their research.
DOI: PLOS Computational Biology, 2022. 10.1371/journal.pcbi.1009869 (About DOIs).