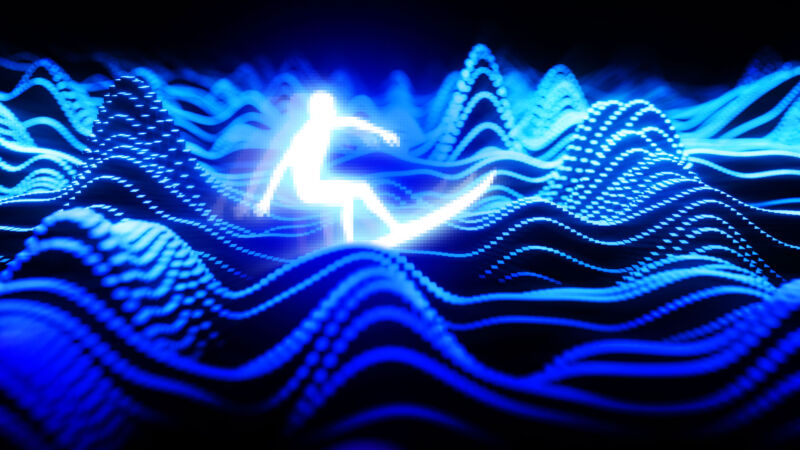
Aurich Lawson / Getty Images
Inspired by the action of pulsed lasers, scientists from France and Japan have developed an acoustic counterpart that allows the precise and controlled transfer of individual electrons between quantum nodes.
Riding the waves
An electron’s spin can serve as the basis for creating qubits – the basic unit of information of quantum computing. To process or store that information, the information in qubits may need to be transported between quantum nodes in a network.
One possibility is to transport the electrons themselves, something that can now be done by letting them ride on sound waves. “We first demonstrated it more than 10 years ago,” said lead researcher Christopher Bauerle of the Grenoble-based Institute Néel.
However, this technique had a significant drawback. Like any wave, a sound wave takes on a sinusoidal shape, made up of many maxima and minima, making it difficult to predict the electron’s location.
Bauerle and his team have now circumvented this problem by designing a wave that has a single minimum or a single maximum. “Using a technique called Fourier synthesis, we superimposed many waves of different frequencies in such a way that there was only one minimum or one maximum, depending on whether you apply positive or negative voltage,” he said.
Bauerle compares these concentrated sound waves with laser pulses. “If you want to take time-resolved measurements, you stimulate a system with a short laser pulse. We use a similar technique in our system with sound. Because we have a focused acoustic pulse, we know exactly what time the electron will arrive at a node,” he said.
Paulo Santos, a Berlin-based nanoelectronics expert at the Paul Drude Institute for Solid State Electronics, likens the technique to a surfer riding a wave. “Just like a surfer is transported by a wave in an ocean, the electron qubit rides the surface acoustic wave to move in the quantum network,” noted Santos, who was not part of the study.
Making waves
To generate these sound waves, a chip with quantum nodes embedded in a gallium arsenide crystal was coupled to two gold-plated electrodes deposited on a piezoelectric substrate. An electric field is generated by applying an alternating voltage to these electrodes. The varying electric field deforms the piezoelectric material and generates surface acoustic waves. They are accompanied by a moving electric field (generated by the inverse piezoelectric effect) that helps to transport the electrons.
Bauerle listed several advantages of this system, which operates at temperatures between 20 milliKelvin to one Kelvin. “The electrons are transported between the nodes at the speed of sound (3,000 m/s). This, together with the precise and controlled way of electron transmission, allows us to manipulate the quantum information in real time. If you compare it with In the photonic quantum system, the manipulations must be done ahead of time because information is sent at the speed of light, which is too fast for real-time manipulation,” he said.
In addition, this technique can potentially be scaled up due to the large size of the waveform. “A single acoustic wave can simultaneously transport electrons from several quantum nodes,” Bauerle said, adding that they achieved 99.4 percent transmission efficiency during their experiments.
According to Santos, the unique ability of this technique to accurately transport qubits and manipulate them on-the-fly on a chip could have a number of different applications in the future. “The next big step is to demonstrate the entanglement of these flying qubits. The other big effort will be to transfer this technology from gallium arsenide to other materials like silicon.”
However, he added that it could be years before practical applications based on this research are seen.
Santos stressed that electron spins are just one of many approaches to processing quantum information; other options include photons, superconducting qubits, and cold atoms. He pointed out that photon qubits will remain a common approach in quantum systems.
“More people are working on photon-based quantum processing because a huge infrastructure already exists. For example, silicon-based chips also have integrated optics. The ‘electron surfing’ technique is compatible with on-chip integration and can benefit from these developments,” said he, suggesting that progress in one may help in the other.
Physical Assessment X, 2022. DOI: 10.1103/PhysRevX.12.031035
Dhananjay Khadilkar is a journalist from Paris.