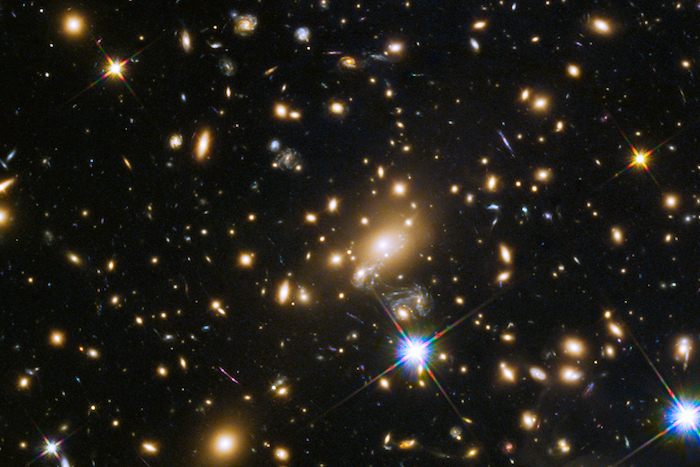
Anyone who has ever measured something twice, such as the width of a doorway, and received two different answers knows how annoying it can be. Now imagine you’re a physicist, and what you’re measuring tells us something fundamental about the universe. There are a number of examples like this – for example, we can’t get measurements to agree on how long neutrons survive outside atomic nuclei.
Few of these are more fundamental to the behavior of the Universe than disagreements over what is called the Hubble constant, a measure of how fast the Universe is expanding. We measured it using information in the cosmic microwave background and obtained one value. And we measured it using the apparent distance of objects in the current universe and we got a value that differs by about 10 percent. As far as anyone knows, there’s nothing wrong with either measurement, and there’s no obvious way to get them to agree.
Now researchers have managed to make a third, independent measure of the expansion of the Universe by tracking the behavior of a gravitationally lensed supernova. When the lens was first discovered, it had captured four images of the supernova. But a fifth appeared some time later, and that time lag is influenced by the expansion of the universe – and thus the Hubble constant.
Inconsistent constant
The Hubble constant is a measure of the expansion of the universe, as you can see from the units, which are kilometers per second per Megaparsec. So every second, every megaparsec of the universe expands by a certain number of kilometers. Another way to think about this is in terms of a relatively stationary object at a Megaparsec distance: every second it gets several kilometers further away.
How many kilometres? That’s the problem here. Measurements of the cosmic microwave background using the Planck satellite yielded a value of 67 km/s Mpc. Those performed by tracking distant supernovae produce a value of 73 km/s Mpc. We’re not sure why those measurements should differ, or if there’s a technical issue with one of them that we haven’t identified yet. But it is considered an important unsolved problem.
The new work includes a third way of measuring distance that is independent of the other two. It is based on gravitational lensing, where the distortion in space-time caused by a massive object acts as a lens to magnify an object in the background. Because these are not perfect optical quality lenses, there are often some distortions and imperfections. This causes the light from the background object to take different paths to Earth, and so a single object can appear in different locations around the lens.
On a cosmological scale, those paths may also require light to travel very different distances to reach Earth. And since light travels at a finite speed, that means we can look at a single object as it was in a different way time. For example, last year researchers identified a single image from the Hubble Space Telescope that captured a supernova as it was at three different times after the explosion.
The new work focuses on a similar specimen, a supernova first identified in 2014 and now named SN Refsdal after the astronomer who first proposed using lens explosions to make measurements. When first discovered, distant SN Refsdal was photographed by a cluster of galaxies called MACS J1149.6+2223, which created four different images of it. But studies of the lens formed by MACS J1149.6+2223 quickly showed that it would create an additional image about a year later.
Those predictions turned out to be correct. Images taken in late 2015 identified the fifth image of the event taken by the gravitational lens.